Antiproliferative, Proapoptotic, and Potential Antifibrotic Effects of α-Mangostin in Fibroblasts from Keloid Lesions and Normal Skin
Kevin L. McFarland1, Jennifer M. Hahn, Kelly A. Combs, and Dorothy M. Supp
VISUAL ABSTRACT
ABSTRACT
Keloids are disfiguring lesions that result from an abnormal wound healing process. Despite the availability of numerous therapeutic options, keloids remain challenging to treat and often recur after therapy. α-Mangostin, a natural xanthone isolated from the fruit of the Mangosteen tree, has been used for centuries in many Southeast Asian nations for medicinal purposes, and has gained attention more recently due to its anti-inflammatory, antimicrobial, and antioxidant properties, with numerous studies suggesting possible anticarcinogenic activities. Hypothetically, α-mangostin may have therapeutic value for keloid suppression. To investigate this hypothesis, the effects of α-mangostin on fibroblast proliferation, apoptosis, and gene expression in vitro were analyzed. Dermal fibroblasts were isolated and cultured from normal human skin and excised keloid lesions (3 donors each), and were treated with multiple doses of α-mangostin in vitro. Dose-dependent decreases in proliferation in keloid and normal fibroblasts were observed following treatment with α-mangostin. The α-mangostin treated fibroblasts displayed significantly increased expression of C/EBP homologous protein (CHOP), which mediates endoplasmic reticulum stress-induced apoptosis, suggesting increased apoptosis. In addition, numerous changes in gene expression were observed in α-mangostin-treated keloid fibroblasts, including decreased expression of collagen type I alpha 1 chain (COL1A1) and increased expression of matrix metalloproteinase 1 (MMP1), MMP3, and MMP13. Secretion of pro-collagen I was decreased, and secretion of MMP1 and MMP3 were increased, in α-mangostintreated fibroblasts. The results suggest that α-mangostin may exhibit antiproliferative, proapoptotic, and antifibrotic activities in keloid and normal fibroblasts. Thus, this study suggests that further investigation is warranted to fully explore the therapeutic potential of α-mangostin for suppression of keloid development.
Introduction
Keloids are fibroproliferative lesions that can result from an abnormal wound healing response. Although historically considered an extreme form of pathological scarring, the term “keloid disorder” has been proposed to distinguish these lesions from other types of scars, such as hypertrophic scars.1,2 Keloids are characterized by excessive and abnormal deposition of collagen, with an elevated ratio of type I to type III collagen.3 Unlike hypertrophic scars, which are raised but limited to the original wound area, keloids tend to bulge over the original wound margin and can continue to increase in size indefinitely.4 Keloids are relatively common, although the incidence varies among different populations, with increased risk of keloid formation in individuals of African, Asian, and Hispanic descent compared with those of European ancestry.1,2,5 Keloids are disfiguring and can cause pain, itching, decreased range of motion, and impaired psychosocial well-being.6-9 Keloids are extremely resistant to treatment, usually recurring even after surgical excision.1 There are many different treatment modalities for keloids, but most have limited long-term success, and highquality evidence supporting clinical efficacy is lacking for most therapies.1,10,11 Improved therapeutic interventions are needed to suppress keloid pathology and alleviate patient morbidity.
The molecular mechanisms leading to keloid formation remain incompletely understood, despite numerous studies reporting gene expression abnormalities in keloids and keloid-derived cells.12-15 The fact that keloids have an increased prevalence in certain populations, and tend to run in families, suggests a genetic predisposition.16 Although numerous chromosomal loci have been associated with keloid risk, no causative gene has been identified to date, and it is likely that keloid susceptibility is a multigenic trait.16,17 Keloid fibroblasts exhibit increased proliferation, decreased apoptosis, increased expression of type I collagen, and exaggerated responses to transforming growth factor beta 1 (TGF-β1) signaling.3,18-20 In addition, keloid fibroblasts exhibit increased expression of proinflammatory cytokines and altered regulation of genes in multiple signaling pathways, including TGF-β1 and WNT/β-catenin pathways. 13-15,21 Agents that reverse this fibrotic phenotype are expected to have potential therapeutic value for keloid suppression. There has been increasing interest in recent decades in the medicinal uses of natural, botanical dietary supplements, due in part to their perceived healthpromoting qualities.22 Many botanical supplements have demonstrated medicinal properties, such as anti-inflammatory, anti-infective, and anti-oxidant properties, and there has been a great deal of research into potential therapeutic applications of phytochemicals. One plant that has a long history of medicinal use is Garcinia mangostana, also known as mangosteen, a tropical tree native to Southeast Asia. The fruit of the mangosteen tree has been used for centuries as a medicinal agent, particularly for treatment of wounds and skin infections, as well as abdominal pain, diarrhea, dysentery, and ulcers.23,24 The pericarp of the fruit is rich in xanthones, a class of polyphenolic compounds with numerous biological activities; among these, α-mangostin is a major constituent.24 Because α-mangostin has been reported to exhibit numerous pharmacological properties, it has been explored as an anti-cancer agent. Evidence from in vitro and preclinical in vivo studies suggests that α-mangostin has anticarcinogenic activities affecting tumor initiation, promotion, and progression.25 α-Mangostin has potent antioxidant activities, which include scavenging of free radicals and modulation of oxidative stress-related enzymes.23,25 In addition, α-mangostin has antiproliferative and proapoptotic activities.25 For example, α-mangostin reduced proliferation via cell cycle arrest in a human colon cancer cell line, in part through downregulation of cyclin protein levels, and induced apoptosis in human leukemia cell lines and a human osteosarcoma cell line.26- 29 Anti-inflammatory activity of α-mangostin has been demonstrated in multiple human cell lines from different tissues; for example, α-mangostin reduced expression of the proinflammatory mediators tumor necrosis factor alpha (TNFα) and interleukin 8 (IL8) in HepG2 hepatocyte carcinoma cells, Caco-2 colorectal adenocarcinoma cells, and HT-29 colon cancer cells.30 Antifibrotic activities have been demonstrated in hepatic stellate cells in vitro, suggesting therapeutic potential for suppression of liver fibrosis.31,32 For example, treatment of LX2 hepatic stellate cells with α-mangostin decreased proliferation, TGF-β1 secretion, and decreased expression of collagen type I alpha 1 chain (COL1A1), alpha smooth muscle actin, and phosphorylated SMAD family member 3 (SMAD3), considered markers of fibrogenesis.31,32
Because of the antiproliferative, anti-inflammatory, proapoptotic, and anti-fibrotic activities of α-mangostin that were previously described, it may represent an attractive new therapeutic for suppression of keloid scarring. To begin to explore this possibility, we investigated the effects of α-mangostin in keloid-derived cells in vitro. Fibroblasts cultured from keloid lesions and normal human skin were treated with α-mangostin; proliferation was quantified, and expression levels of fibrotic markers and other genes implicated in keloid pathology were analyzed.
METRICS
Antiproliferative, Proapoptotic, And Potential Antifibrotic Effects Of Α-Mangost In In Fibroblasts
Kevin L. McFarland
University of Cincinnati College of Medicine, Department of Surgery, Cincinnati, Ohio, USA
Jennifer M. Hahn
University of Cincinnati College of Medicine, Department of Surgery, Cincinnati, Ohio, USA
Kelly A. Combs
University of Cincinnati College of Medicine, Department of Surgery, Cincinnati, Ohio, USA
Dorothy M. Supp
(a) University of Cincinnati College of Medicine, Department of Surgery, Cincinnati, Ohio, USA
(b) Shriners Hospitals for Children, Scientific Staff, Dayton, Ohio, USA
(c) Cincinnati Children’s Hospital Medical Center, Center for Stem Cell & Organoid Medicine (CuSTOM), Cincinnati, Ohio, USA,
Address for Correspondence
Dorothy M. Supp, Ph.D. Department of Surgery University of Cincinnati College of Medicine
231 Albert Sabin Way PO Box 670558
Cincinnati, Ohio, 45267-0558, USA
Tel: (513) 558-4748
dorothy.supp@uc.edu
Running title
Effects of a-Mangostin in Keloid and Normal Fibroblasts
Word Count
3,454; Abstract: 258 words
MATERIALS AND METHODS
Primary cell culture
Human tissue samples were obtained with approval of the University of Cincinnati Institutional Review Board, in accordance with Declaration of Helsinki principals. All tissue samples were assigned donor strain identification numbers upon receipt in the laboratory (Table 1). Two of the excised keloid lesions (donor strains 830 and 934) were collected with informed consent from patients undergoing elective surgeries at Shriners Hospitals for Children – Cincinnati. The remaining keloid sample (donor strain 961) and three normal skin samples were collected as de-identified surgical discard tissue from patients undergoing elective plastic surgery procedures at the University of Cincinnati Medical Center. For collection of de-identified normal skin, the Institutional Review Board determined that this activity did not meet the definition of human subjects research; therefore, patient consent was not required. Patient demographic information is presented in Table I.
Primary cultures were established as previously described.15,33 Briefly, tissue samples were trimmed to remove subcutaneous fat, cut to strips measuring approximately 0.5 cm wide and 1-2 cm long, and incubated overnight at 4° C in Dispase II (MilliporeSigma, St. Louis, MO). Following removal of the epidermis, the dermal strips were finely minced and incubated in collagenase (Worthington Biochemical Corp., Lakewood, NJ) for 1-2 hours with occasional mixing. Fibroblasts were pelleted by mild centrifugation (1,000 rpm, 7 minutes, at 4° C), rinsed with culture media, and inoculated into flasks. Culture media consisted of Dulbecco’s Modified Eagle Medium (DMEM; Thermo Fisher Scientific, Waltham, MA) supplemented with 10 ng/ml epidermal growth factor (PeproTech, Rocky Hill, NJ), 5 µg/ml insulin (Thermo Fisher), 0.5 µg/ml hydrocortisone (MilliporeSigma), 0.1 mM ascorbic acid-2-phosphate (MilliporeSigma), 4% Fetal Bovine Serum (FBS; Thermo Fisher), and 1X Antibiotic-Antimycotic Solution (Thermo Fisher). Culture media was exchanged every other day and cells were passaged when they reached 80-90% confluence (5-6 days). Cells were utilized for experiments at passage two or three.
Table I. Demographic data for donors of tissue used for primary cell culture. | |||||
Strain # | Tissue Type | Donor Sex | Donor Age | Donor Ethnicity* | Body Site |
918 | Normal Skin | Female | 25 | African American | Breast |
927 | Normal Skin | Female | 31 | African American | Breast |
979 | Normal Skin | Female | 19 | African American | Thigh |
830 | Keloid | Male | 17 | African American | Ear |
934 | Keloid | Female | 15 | African American | Ear |
961 | Keloid | Female | 26 | African American | Back |
*Donor ethnicity was self-reported. |
Proliferation Assay
α-Mangostin (MilliporeSigma) was dissolved in dimethyl sulfoxide to make a 10.0 mM stock solution; this was further diluted in culture media to obtain the desired concentrations. Fibroblast proliferation was measured using an MTT (3-(4,5-dimethylthiazol-2- yl)-2,5-diphenyltetrazolium bromide) assay as detailed elsewhere.34 Normal and keloid fibroblasts (N = 3 strains each) at passage 2 were inoculated into wells in 24- well plates at 1.0 X 104 /cm2 in duplicate, and 48 hours later α-mangostin (MilliporeSigma) was added to the media to yield final concentrations of 0.0 µm (vehicle control), 1.0 µm, 2.5 µm, 5.0 µm, 7.5 µm, and 10.0 µm. Cells were incubated for 4, 8, 12, or 24 hours. For the 24 hour incubation, the medium was changed to fresh medium containing α-mangostin or vehicle at 12 hours, and the incubation was continued for another 12 hours, for a total incubation time of 24 hours. This was done because previous reports indicated that α-mangostin has variable stability in different types of culture medium.30 Gutierrez-Orozco et al. found that only about half of α-mangostin activity was lost after incubation in DMEM for 12 hours; however, the activity was increased to near starting levels by addition of 10% FBS to the culture media.30 As the DMEM culture media in the current experiment contained 4% FBS, the media was changed after 12 hours with fresh addition of α-mangostin to compensate for any potential loss of activity in the culture media. At the end of the incubation period, the MTT assay (MP Biomedical, Santa Ana, CA) was performed, and absorbance was measured at a wavelength of 570 nm. Absorbance provides an index of cellular metabolic activity, which is proportional to cell number. The absorbance values were normalized to levels of vehicle-treated cells of the same donor strain at the same time point (controls), and data are expressed as the mean percent of control for all three cell strains for each fibroblast type (normal or keloid).
Gene Expression Analysis
Normal and keloid fibroblasts (3 donor strains each) at passage 3 were inoculated in duplicate into 6-well plates at a density of 1.0 X 104 /cm2 . After 48 hours, cells were treated with vehicle or α-mangostin at 5 µM. The media was changed and fresh α-mangostin (or vehicle) was added at 12 hours, and cells were harvested at 24 hours for isolation of total RNA using the Qiagen RNAeasy mini kit (Qiagen, Inc., Valencia, CA). The SuperScript VILO cDNA Synthesis Kit (Thermo Fisher) was used for preparation of cDNA. Real-time quantitative polymerase chain reaction (qPCR) was used for analysis of gene expression. Amplification was performed with genespecific primers (RT2 qPCR Primer Assays; Qiagen) and RT2 SYBR Green qPCR Mastermix (Qiagen) using the StepOne Plus Real Time PCR System (Thermo Fisher). Technical triplicates of the qPCR amplification were performed in addition to biological replicates. Genes analyzed included COL1A1, matrix metalloproteinase 1 (MMP1), matrix metalloproteinase 3 (MMP3), matrix metalloproteinase 13 (MMP13), hyaluronan synthase 2 (HAS2), periostin (POSTN), transforming growth factor beta 1 (TGFB1), vascular endothelial growth factor (VEGF), cyclin D1 (CCND1), cyclin D2 (CCND2), interleukin 6 (IL6), serpin family H member 1 (SERPINH1), which is also known as heat shock protein 47 (HSP47), GLI Family Zinc Finger 1 (GLI1), and C/EBP homologous protein (CHOP), also known as DNA damage-inducible transcript 3 (DDIT3) and as growth arrest and DNA damage-inducible protein 153 (GADD153). Expression levels were referenced to the glyceraldehyde 3-phosphate dehydrogenase (GAPDH) gene using the comparative 2-∆∆Ct method.35 A mean expression level was calculated for each pair of duplicate samples for each group (control or α-mangostin-treated) and cell strain, and the relative expression in α-mangostintreated cells was normalized to the level in untreated cells of the same strain, providing a fold change expression level for each cell strain. Data for normal and keloid fibroblasts are presented as the mean for each cell type (N = 3 donor strains) + standard error of the mean (SEM).
Analysis of Protein Secretion
Levels of MMP1, MMP3, and pro-collagen type I in culture media were determined using Enzyme-Linked Immunosorbent Assays (ELISAs). Cells were cultured and treated with α-mangostin or vehicle (control) for 24 hours as described for analysis of gene expression, and media was harvested and stored frozen at -20° C until used in ELISAs. MMP1 and MMP3 levels in culture media were measured using the Human Pro-MMP-1 Quantikine ELISA Kit and the Human Total MMP-3 Quantikine ELISA Kit (R&D Systems, Minneapolis, MN) according to the manufacturer’s instructions. Collagen type I was measured using the Human ProCollagen I alpha 1 ELISA Kit (Abcam, Inc., Cambridge, MA) according to manufacturer’s instructions.
Statistical analysis
Statistical analysis was performed and data plots generated using SigmaPlot 13 (Systat Software, Inc., San Jose, CA). Pairwise comparisons were analyzed using the t-test. Multiple comparisons over time (proliferation assay) for each cell type were analyzed using One Way Repeated Measures Analysis of Variance. Differences were considered statistically significant at P < 0.05.
Results
Treatment of normal or keloid fibroblasts with increasing concentrations of α-mangostin (1.0-10.0 µM) resulted in a dose-dependent decrease in proliferation over time compared with vehicle-treated control cells (Figure 1). Significant differences among varying α-mangostin concentrations over time were observed. There were no differences in sensitivity to α-mangostin between normal and keloid fibroblasts, except for the highest concentration at 24 hours; in 10 µM α-mangostin, there was a 98.56% reduction in proliferation in normal fibroblasts, but a 99.39% reduction in keloid fibroblasts (P < 0.05).
To determine whether apoptosis contributes to the reduction in proliferation of normal and keloid fibroblasts, gene expression of CHOP, which is induced in response to endoplasmic reticulum (ER) stress-induced apoptosis36, was measured by qPCR. A significant increase in CHOP expression was observed in both normal and keloid fibroblasts treated with α-mangostin (Figure 2), suggesting increased apoptosis due to α-mangostin treatment. No significant differences in basal CHOP expression were observed between untreated normal and keloid fibroblasts (data not shown).
To determine the effects of α-mangostin on gene expression, normal and keloid fibroblasts were treated with 5.0 µM α-mangostin, the middle concentration tested in the proliferation assay. Expression was analyzed for genes previously implicated in keloid disorder, or genes affected by α-mangostin treatment in other cell types. These include genes involved in extracellular matrix (ECM) deposition and remodeling: COL1A1, HAS2, POSTN, and MMP13, which are reportedly increased in keloid fibroblasts compared with normal fibroblasts, and MMP1 and MMP3, which are decreased in keloid versus normal fibroblasts.3,15,37,38 Expression of COL1A1 and HAS2 was reduced in α-mangostin treated fibroblasts; COL1A1 reduction was modest but statistically significant in keloid fibroblasts, and the reduction in HAS2 was significant in normal fibroblasts (Figure 3). MMP1, MMP3, and MMP13 were all increased in fibroblasts treated with α-mangostin. The increases in MMP1 and MMP13 expression were significant in both normal and keloid fibroblasts, and the increase in MMP3 was significant only in keloid fibroblasts. Surprisingly, POSTN was increased in fibroblasts, and this increase was statistically significant in keloid fibroblasts.
Growth factors TGF-β1, a profibrotic growth factor that is increased in keloid lesions compared with normal skin39, and VEGF, an angiogenic growth factor that is similarly expressed in keloid versus normal fibroblasts but upregulated by hypoxia in keloid fibroblasts40, were also examined. No significant changes in TGF-β1 were observed, but VEGF was significantly increased in normal fibroblasts. VEGF was increased in keloid cells as well, but the difference between α-mangostin-treated and control cells was not statistically significant (Figure 3). Treatment with α-mangostin had no statistically significant effects on expression of cyclin CCND1, however CCND2 was significantly increased in normal and keloid fibroblasts treated with α-mangostin. The serine protease SERPINH1, which was previously reported to be increased in keloid lesions compared with normal skin, was increased in response to α-mangostin, with a significant increase observed in normal fibroblasts (Figure 3).41 Cytokines CCL2, also referred to as monocyte chemoattractant protein 1 (MCP1), and IL6, were previously shown to be upregulated in keloid lesions and keloid fibroblasts, respectively, compared with normal skin and skin-derived fibroblasts.15,42,4 Expression of CCL2 was significantly downregulated by α-mangostin treatment in both normal and keloid fibroblasts. Interestingly, IL6 expression was increased in both normal and keloid fibroblasts, and this increase was statistically significant in keloid fibroblasts (Figure 3). A previous report found that the transcription factor GLI1, which functions in the Sonic hedgehog signaling pathway, was downregulated in pancreatic cancer stem cells in response to α-mangostin.29 In contrast, GLI1 expression was significantly increased in normal and keloid fibroblasts treated with α-mangostin (Figure 3).
Because ECM deposition is regulated at multiple levels, including the post-transcriptional level, we measured levels of pro-collagen I, MMP1, and MMP3 secreted into the culture media of normal and keloid fibroblasts. Treatment with α-mangostin resulted in significantly decreased levels of pro-collagen I, and significantly increased levels of MMP1 and MMP3, in both normal and keloid fibroblasts (Figure 4).
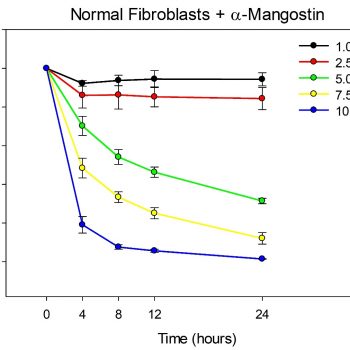
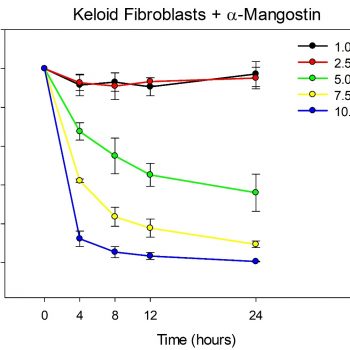
FIGURE 1: Proliferation is reduced in fibroblasts treated with α-mangostin. Plotted are mean values (+/- SEM) for normal fibroblasts (left) and keloid fibroblasts (right) treated with varying concentrations of α-mangostin, as indicated (N = 3 donor strains/cell type). Values are expressed as a percent of untreated controls for each time point. For both normal and keloid fibroblasts, there was a statistically significant interaction between dose and time (P < 0.001). The values for 1.0 µM (black line) and 2.5 µM (red line) were not significantly different than each other, but were significantly greater than all other concentrations at 4-24 hours. For 5.0 µM (green line), 7.5 µM (yellow line), and 10 µM (blue line) α-mangostin, the differences among concentrations were statistically significant at all time points, except for the comparison between 7.5 µM and 10.0 µM at 24 hours. There were no significant differences between normal and keloid fibroblasts, except for 10.0 µM α-mangostin at 24 hours; at this concentration and time, the mean for keloid fibroblasts (0.61%) was significantly lower (P = 0.025) than for normal fibroblasts (1.44%)
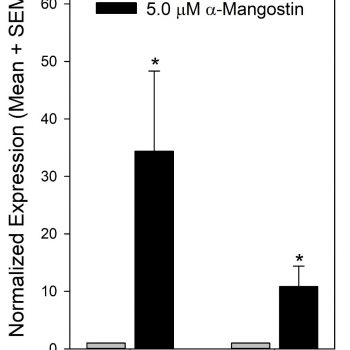
FIGURE 2: Induction of C/EBP homologous protein (CHOP) in fibroblasts by α-mangostin treatment. Plotted are mean gene expression levels (+ SEM) for normal and keloid fibroblasts, as indicated, treated with vehicle (grey bars) or α-mangostin (black bars). The expression levels in α-mangostin-treated cells were normalized to mean expression in untreated fibroblasts (N = 3 donor strains/group). Significant increases in CHOP expression were observed (*) in α-mangostin-treated fibroblasts (P < 0.05 vs. control), suggesting induction of apoptosis.
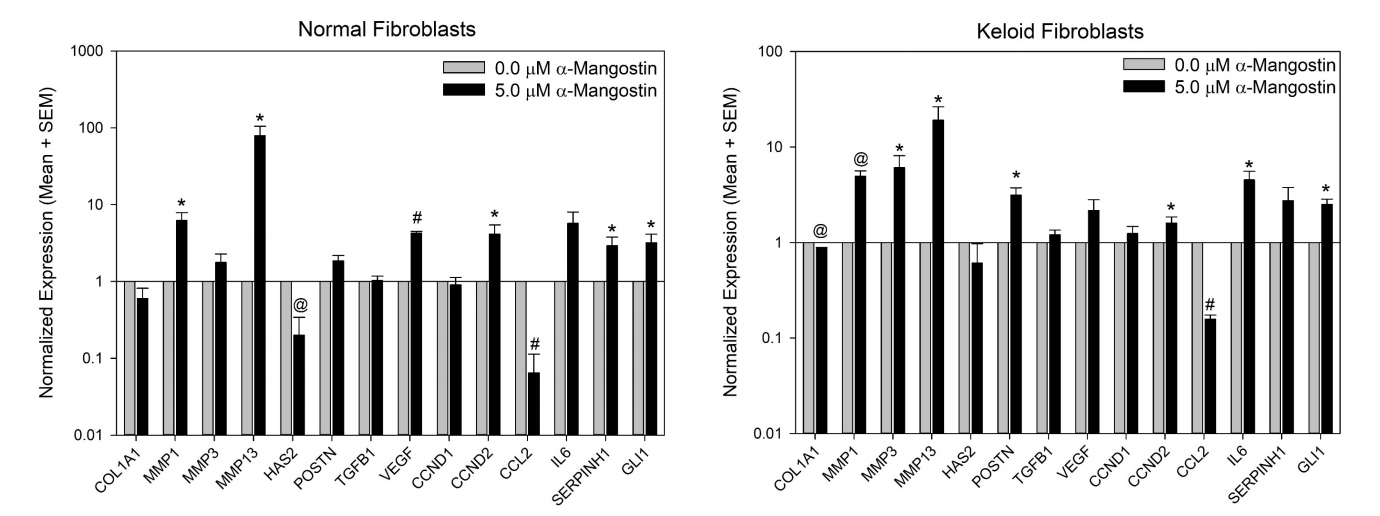
FIGURE 3: Altered gene expression in fibroblasts treated with α-mangostin. Plotted are mean gene expression levels (+ SEM) for normal fibroblasts (left) and keloid fibroblasts (right) treated with vehicle (grey bars) or α-mangostin (black bars). The expression level for each gene is normalized to mean expression in untreated fibroblasts (N = 3 donor strains/group). Statistically significant expression differences are indicated by symbols: P < 0.05 vs. control (*); P < 0.01 vs. control (@); and P < 0.001 vs. control (#).
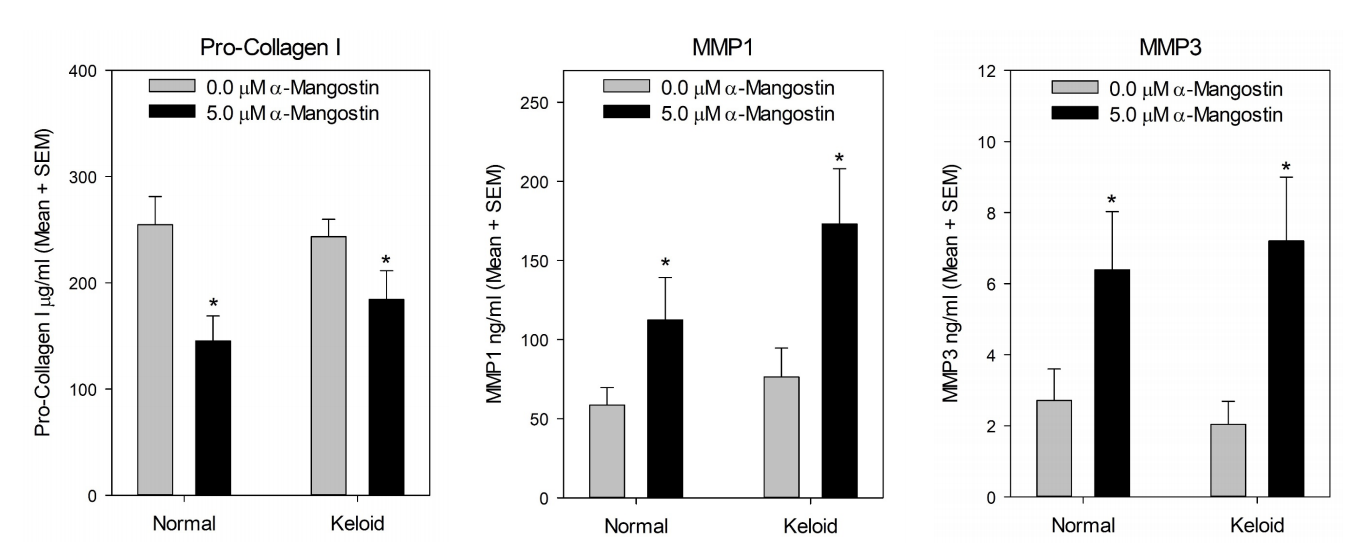
FIGURE 4: Altered secretion of collagen type I, MMP1, and MMP3 in fibroblasts treated with α-mangostin. Plotted are mean levels of pro-collagen I (left), MMP1 (center), and MMP3 (right) measured in culture media from control (grey bars) and α-mangostin-treated (black bars) normal and keloid fibroblasts, as indicated (N = 3 donor strains/group). Statistically significant differences are indicated by asterisks (*); P < 0.05.
DISCUSSION
Keloids are disfiguring lesions resulting from an abnormal wound healing process. In this study, we demonstrate for the first time the antiproliferative, proapoptotic, and potential antifibrotic effects of α-mangostin in keloid fibroblasts and normal human dermal fibroblasts. One prominent feature of keloids is excessive and abnormal deposition of collagen, which is thought to result from an imbalance of collagen production and degradation.44 The opposing effects of α-mangostin on the levels of MMPs and collagen type I observed in the current study suggest that it may have potential therapeutic value for suppression of collagen deposition in keloid lesions.
Different parts of the G. mangostana plant have been used medicinally for centuries in Southeast Asia, with demonstrated antimicrobial and anti-inflammatory properties.23,24 These properties would be expected to enhance wound healing, but as suggested here, it may act directly on fibroblasts to regulate wound healing processes including fibroblast proliferation, ER stressinduced apoptosis, and gene expression. A previous study analyzed the in vitro wound healing properties of α-mangostin, and found that α-mangostin treatment caused an increase in proliferation of murine 3T3 fibroblasts.45 Although this appears to contrast with the current study, those experiments were performed in an immortalized mouse embryonic fibroblast cell line45, which may be expected to behave differently than primary human fibroblasts isolated from adult skin or keloids. In addition, that study found increased migration in α-mangostin-treated murine 3T3 cells, as measured by an in vitro wound healing assay, suggesting a possible basis for the traditional use of α-mangostin as a therapy for chronic wounds.24 We attempted to investigate the effects of α-mangostin using a similar in vitro wound healing assay; however, we encountered technical difficulties due to detachment of the α-mangostin-treated fibroblasts in an in vitro scratch assay and were thus unable to quantify migration (data not shown). The previous report found enhanced migration in 3T3 cells treated with 1 µg/ml α-mangostin, which is equivalent to about 2.4 µM, roughly half the concentration tested in our study. As we found that 2.5 µM α-mangostin had relatively little effect on fibroblast proliferation, this concentration may have yielded different results, but we chose to examine a dose that exhibited anti-proliferative effects, as might be used therapeutically. Further studies will be required to investigate potential effects on α-mangostin on keloid and normal dermal fibroblast migration and invasion.
In other cellular contexts, α-mangostin has been shown to inhibit invasive activity; for example, in melanoma and squamous cell carcinoma cell lines, α-mangostin inhibited in vitro cell motility and invasion.46 The antitumorigenic properties of α-mangostin include inhibition of growth, reduction of invasion, mitigation of oxidative damage, induction of apoptosis, and suppression of angiogenesis.25 In addition, when combined with clinical chemotherapy drugs, such as 5-fluorouracil, it was found to increase the efficacy of those drugs while decreasing toxic side effects.47 This raises the interesting possibility of using α-mangostin as part of a combination therapy with other drugs currently used to suppress keloid growth.
In spite of the known anti-inflammatory properties of α-mangostin, we found that it increased expression of IL6, a potent inflammatory cytokine that is increased in keloid fibroblasts compared with normal fibroblasts.15 However, CCL2 was significantly decreased in α-mangostin treated fibroblasts, which could serve to reduce inflammation and perhaps decrease fibroblast proliferation as well. CCL2 was found to be increased in keloid lesions and in CD14+ monocytes isolated from keloids; co-culture of these keloid-derived monocytes with fibroblasts led to increased fibroblast proliferation, which was dependent on CCL2. 42 This suggests CCL2 has a role in increased proliferation of keloid fibroblasts.42 We also observed differing effects of α-mangostin on expression of HAS2, which was decreased in response to α-mangostin in normal fibroblasts, and POSTN, which was increased by α-mangostin in keloid fibroblasts. HAS2 and POSTN have demonstrated pro-fibrotic activities48-51, and are both increased in keloid versus normal fibroblasts.15 A more comprehensive investigation of the effects of α-mangostin will be required to understand how the various gene expression changes act in concert to regulate cellular behavior and overall phenotype. In addition, because the current study analyzed expression of CHOP, a marker ER stress-induced apoptosis36, it will be important in future studies to investigate the effects of α-mangostin on other apoptotic pathways. Further studies in additional relevant cell types, such as keratinocytes, and in more complex organotypic models, will help us to better understand the potential therapeutic benefits of α-mangostin for suppression of keloid scarring.
A limitation of the current study is that the analysis was performed using fibroblasts isolated from just three normal skin donors and three keloid donors, including two with keloids of the ear. Several of the observed changes in gene expression indicated trends but did not reach statistical significance. Although the statistical analyses were sufficiently powered to detect differences in proliferation and expression of some genes, there was sufficient variability among donor cell strains for other genes to reduce the statistical power. It is possible that analysis of increased donor cell strains may reveal statistically significant differences that were not detected in the current study. Analysis of cells from a large variety of donors, including donors of varying ethnicities and keloids from different body sites, will be required to confirm the validity of the results reported here. In our experience, there can be tremendous variability among cells isolated from different donors in expression levels of some genes, whereas other genes are consistently overexpressed or underexpressed in keloid versus normal cells.15 This is consistent with the complex genetics of keloid disorder, which likely involves multiple genes as well as interactions with environmental factors.17,52 This suggests that a combination of therapeutic interventions, perhaps involving α-mangostin, may be required to effectively suppress keloid formation.
ACKNOWLEDGEMENTS
The authors thank Dr. Elizabeth Dale, Dr. W. John Kitzmiller, Dr. Petra Warner, and the operating room staff at the University of Cincinnati Medical Center and Shriners Hospitals for Children – Cincinnati for their assistance in collection of surgical discard tissue for use in these experiments. In addition, we thank Laura Fowler and Judy Nelson for obtaining informed consent from keloid patients, and we thank the patients for generously donating their tissue samples for this research. This study was supported by Medical Research Grant #85500-CIN15 from the Shriners Hospitals for Children.
AUTHORSHIP CONTRIBUTIONS
DMS conceived of the study, provided funding for the study, performed statistical data analyses and wrote the manuscript. KLM, JMK, and KAC isolated and cryopreserved primary cells from tissue samples. KLM performed cell culture experiments and data analysis, and helped write and edit the manuscript. JMK and KAC assisted with cell culture experiments and helped write and edit the manuscript.
DISCLOSURE OF CONFLICTS OF INTEREST
The authors have no financial or other conflicts of interest to disclose.
References
1. Uitto J, Tirgan MH. Clinical challenge and call for research on keloid disorder: meeting report from The 3rd International Keloid Research Foundation Symposium, Beijing 2019. J Invest Dermatol 2020, 140(3):515-8.
2. Limandjaja GC, Niessen FB, Scheper RJ, Gibbs S. The keloid disorder: heterogeneity, histopathology, mechanisms and models. Front Cell Dev Biol 2020, 8:360.
3. Uitto J, Perejda AJ, Abergel RP, Chu ML, Ramirez F. Altered steady-state ratio of type I/III procollagen mRNAs correlates with selectively increased type I procollagen biosynthesis in cultured keloid fibroblasts. Proc Natl Acad Sci USA 1985, 82(17):5935-9.
4. Tsai CH, Ogawa R. Keloid research: current status and future directions. Scars Burns Heal 2019, 5:2059513119868659.
5. Velez Edwards DR, Tsosie KS, Williams SM, Edwards TL, Russell SB. Admixture mapping identifies a locus at 15q21.2-22.3 associated with keloid formation in African Americans. Hum Genet 2014, 133(12):1513-23.
6. Bock O, Schmid-Ott G, Malewski P, Mrowietz U. Quality of life of patients with keloid and hypertrophic scarring. Arch Dermatol Res 2006, 297;433-8.
7. Balci DD, Inandi T, Dogramaci CA, Celik E. DLQI scores in patients with keloids and hypertrophic scars: a prospective case control study. J Dtsch Dermatol Ges 2009, 7(8):688-92.
8. Tan A, Glass DA. Patient-reported outcomes for keloids: a systematic review. G Ital Dermatol Venereol 2019, 154(2):148-65.
9. Bijlard E, Kouwenberg CA, Timman R, Hovius SE, Busschbach JJ, Mureau MA. Burden of keloid disease: a cross-sectional health-related quality of life assessment. Acta Derm Venereol 2017, 97(2):225-9.
10. Leventhal D, Furr M, Reiter D. Treatment of keloids and hypertrophic scars: a meta-analysis and review of the literature. Arch Facial Plast Surg 2006, 8(6):362-8.
11. Durani P, Bayat A. Levels of evidence for the treatment of keloid disease. J Plast Reconstr Aesthet Surg 2008, 61:4-17.
12. Satish L, Lyons-Weiler J, Hebda PA, Wells A. Gene expression patterns in isolated keloid fibroblasts. Wound Repair Regen 2006, 14(4):463-70.
13. Smith JC, Boone BE, Opalenik SR, Williams SM, Russell SB. Gene profiling of keloid fibroblasts shows altered expression in multiple fibrosis-associated pathways. J Invest Dermatol 2007, 128(5):1298-310.
14. Russell SB, Russell JD, Trupin KM, Gayden AE, Opalenik SR, Nanney LB, Broquist AH, Raju L, Williams SM. Epigenetically altered wound healing in keloid fibroblasts. J Invest Dermatol 2010, 130(10):2489-96.
15. Hahn JM, Glaser K, McFarland KL, Aronow BJ, Boyce ST, Supp DM. Keloid-derived keratinocytes exhibit an abnormal gene expression profile consistent with a distinct causal role in keloid pathology. Wound Repair Regen 2013, 21(4):530-44.
16. Glass DA. Current understanding of the genetic causes of keloid formation. J Invest Dermatol Symp Proc 2017, 18(2):S50-S3.
17. Shih B, Bayat A. Genetics of keloid scarring. Arch Dermatol Res 2010, 302(5):319-39.
18. Nakaoka H, Miyauchi S, Miki Y. Proliferating activity of dermal fibroblasts in keloids and hypertrophic scars. Acta Derm Venereol 1995, 75(2):102-4.
19. Luo S, Benathan M, Raffoul W, Panizzon RG, Egloff DV. Abnormal balance between proliferation and apoptotic cell death in fibroblasts derived from keloid lesions. Plast Reconstruct Surg 2001, 107(1):87-96.
20. Bettinger DA, Yager DR, Diegelmann RF, Cohen IK. The effect of TGF-beta on keloid fibroblast proliferation and collagen synthesis. Plast Reconstruct Surg 1996, 98(5):827-33.
21. Igota S, Tosa M, Murakami M, Egawa S, Shimizu H, Hyakusoku H, Ghazizadeh M. Identification and characterization of Wnt signaling pathway in keloid pathogenesis. Int J Med Sci 2013, 10(4):344-54.
22. Benatrehina PA, Pan L, Naman CB, Li J, Kinghorn AD. Usage, biological activity, and safety of selected botanical dietary supplements consumed in the United States. J Trad Comp Med 2018, 8(2):267-77.
23. Pedraza-Chaverri J, Cárdenas-Rodríguez N, Orozco-Ibarra M, Pérez-Rojas JM. Medicinal properties of mangosteen (Garcinia mangostana). Food Chem Toxicol 2008, 46(10):3227-39.
24. Obolskiy D, Pischel I, Siriwatanametanon N, Heinrich M. Garcinia mangostana L.: a phytochemical and pharmacological review. Phytother Res 2009, 23(8):1047-65.
25. Zhang KJ, Gu QL, Yang K, Ming XJ, Wang JX. Anticarcinogenic effects of alpha-mangostin: a review. Planta Medica 2017, 83(3-04):188-202.
26. Matsumoto K, Akao Y, Ohguchi K, Ito T, Tanaka T, Iinuma M, Nozawa Y. Xanthones induce cell-cycle arrest and apoptosis in human colon cancer DLD-1 cells. Bioorg Med Chem 2005, 13(21):6064-9.
27. Matsumoto K, Akao Y, Kobayashi E, Ohguchi K, Ito T, Tanaka T, Iinuma M, Nozawa Y. Induction of apoptosis by xanthones from mangosteen in human leukemia cell lines. J Nat Prod 2003, 66(8):1124-7.
28. Matsumoto K, Akao Y, Yi H, Ohguchi K, Ito T, Tanaka T, Kobayashi E, Iinuma M, Nozawa Y. Preferential target is mitochondria in alpha-mangostin-induced apoptosis in human leukemia HL60 cells. Bioorg Med Chem 2004, 12(22):5799-806.
29. Park SJ, Park BS, Yu SB, Kang HM, Kim HJ, Kim IR. Induction of apoptosis and inhibition of epithelial mesenchymal transition by α-mangostin in MG-63 cell lines. Evid Based Complement Alternat Med 2018, 2018:3985082.
30. Gutierrez-Orozco F, Chitchumroonchokchai C, Lesinski GB, Suksamrarn S, Failla ML. α-Mangostin: anti-inflammatory activity and metabolism by human cells. J Agric Food Chem 2013, 61(16):3891-900.
31. Rahmaniah R, Yuyuntia Y, Soetikno V, Arozal W, Antarianto RD, Louisa M. Alpha mangostin inhibits hepatic stellate cells activation through TGF-β/Smad and Akt signaling pathways: an in vitro study in LX2. Drug Res 2018, 68(3):153-8.
32. Lestari N, Louisa M, Soetikno V, Suwana AG, Ramadhan PA, Akmal T, Arozal W. Alpha mangostin inhibits the proliferation and activation of acetaldehyde induced hepatic stellate cells through TGF-beta and ERK 1/2 pathways. J Toxicol 2018, 2018:5360496.
33. Supp DM, Hahn JM, Glaser K, McFarland KL, Boyce S. Deep and superficial keloid fibroblasts contribute differentially to tissue phenotype in a novel in vivo model of keloid scar. Plast Reconstruct Surg 2012, 129(6):1259-71.
34. Supp DM, Hahn JM, McFarland KL, Glaser K. Inhibition of hyaluronan synthase 2 reduces the abnormal migration rate of keloid keratinocytes. J Burn Care Res 2014, 35(1):84-92.
35. Livak KJ, Schmittgen TD. Analysis of relative gene expression data using real-time quantitative PCR and the 2(-delta delta C(T)) method. Methods 2001, 25:402-8.
36. Hu H, Tian M, Ding C, Yu S. The C/EBP Homologous Protein (CHOP) transcription factor functions in endoplasmic reticulum stress-induced apoptosis and microbial infection. Front Immunol 2018, 9:3083.
37. McFarland KL, Glaser K, Hahn JM, Boyce ST, Supp DM. Culture medium and cell density impact gene expression in normal skin and abnormal scar-derived fibroblasts. J Burn Care Res 2011, 32(4):498-508.
38. Uchida G, Yoshimura K, Kitano Y, Okazaki M, Harii K. Tretinoin reverses upregulation of matrix metalloproteinase-13 in human keloid-derived fibroblasts. Exp Dermatol 2003, 12 Suppl 2:35-42.
39. Peltonen J, Hsiao LL, Jaakkola S, Sollberg S, Aumailley M, Timpl R, Chu ML, Uitto J. Activation of collagen gene expression in keloids: co-localization of type I and VI collagen and transforming growth factor-beta 1 mRNA. J Invest Dermatol 1991, 97(2):240-8.
40. Steinbrech DS, Mehrara BJ, Chau D, Rowe NM, Chin G, Lee T, Saadeh PB, Gittes GK, Longaker MT. Hypoxia upregulates VEGF production in keloid fibroblasts. Ann Plast Surg 1999, 42(5):514-9.
41. Naitoh M, Hosokawa N, Kubota H, Tanaka T, Shirane H, Sawada M, Nishimura Y, Nagata K. Upregulation of HSP47 and collagen type III in the dermal fibrotic disease, keloid. Biochem Biophys Res Commun 2001, 280(5):1316-22.
42. Liao WT, Yu HS, Arbiser JL, Hong CH, Govindarajan B, Chai CY, Shan WJ, Lin YF, Chen GS, Lee CH. Enhanced MCP-1 release by keloid CD14+ cells augments fibroblast proliferation: role of MCP-1 and Akt pathway in keloids. Exp Dermatol 2010, 19(8):e142-e50.
43. Ikeda M, Naitoh M, Kubota H, Ishiko T, Yoshikawa K, Yamawaki S, Kurokawa M, Utani A, Nakamura T, Nagata K, Suzuki S. Elastic fiber assembly is disrupted by excessive accumulation of chondroitin sulfate in the human dermal fibrotic disease, keloid. Biochem Biophys Res Commun 2009, 390(4):1221-8.
44. Gauglitz GG, Korting HC, Pavicic T, Ruzicka T, Jeschke MG. Hypertrophic scarring and keloids: pathomechanisms and current and emerging treatment strategies. Mol Med 2011, 17(1-2):113-25.
45. Siriwattanasatorn M, Itharat A, Thongdeeying P, Ooraikul B. In vitro wound healing activities of three most commonly used thai medicinal plants and their three markers. Evid Based Ccomplement Alternat Med 2020, 2020:6795383.
46. Wang JJ, Sanderson BJ, Zhang W. Significant anti-invasive activities of α-mangostin from the mangosteen pericarp on two human skin cancer cell lines. Anticancer Res 2012, 32(9):3805-16.
47. Lee J, Kang JS, Choi BY, Keum YS. Sensitization of 5-fluorouracil-resistant SNUC5 colon cancer cells to apoptosis by α-mangostin. Biomol Ther 2016, 24(6):604-9.
48. Yamaguchi Y. Periostin in skin tissue and skin-related diseases. Allergol Internat 2014, 63(2):161-70.
49. Li Y, Liang J, Yang T, Monterrosa Mena J, Huan C, Xie T, Kurkciyan A, Liu N, Jiang D, Noble PW. Hyaluronan synthase 2 regulates fibroblast senescence in pulmonary fibrosis. Matrix Biol 2016, 55:35-48.
50. Li Y, Jiang D, Liang J, Meltzer EB, Gray A, Miura R, Wogensen L, Yamaguchi Y, Noble PW. Severe lung fibrosis requires an invasive fibroblast phenotype regulated by hyaluronan and CD44. J Exp Med 2011, 208(7):1459-71.
51. Vollmann EH, Cao L, Amatucci A, Reynolds T, Hamann S, Dalkilic-Liddle I, Cameron TO, Hossbach M, Kauffman KJ, Mir FF, Anderson DG, Novobrantseva T, Koteliansky V, Kisseleva T, Brenner D, Duffield J, Burkly LC. Identification of novel fibrosis modifiers by in vivo siRNA silencing. Mol Ther Nucleic Acids 2017, 7:314-23.
52. Shih B, Garside E, McGrouther DA, Bayat A. Molecular dissection of abnormal wound healing processes resulting in keloid disease. Wound Repair Regen 2010, 18(2):139-53.